Remote Sensing (RS) is a type of geospatial technology that collects samples of emitted and reflected electromagnetic (EM) radiation from terrestrial, atmospheric, and aquatic ecosystems to detect and monitor the physical characteristics of the terrain without physical contact. This method of data collection typically involves aerial (currently exclusively UAVs) and satellite sensors, which are classified as passive or active sensors. Ground sensors (instruments) are used at the local level and to enhance the quality of satellite and aerial data.
In recent years, ground RS sensors have been included in the field of remote sensing, which, in combination with space and aviation as carriers of sensors, allows for a new level of detail in the studied area or object.
Passive sensors respond to external stimuli by collecting radiation that is reflected or emitted by an object or the surrounding space. The most common source of radiation measured by passive remote sensing is reflected sunlight. Popular examples of passive remote sensors include charge-coupled devices, digital photography and video recording, radiometers, hyperspectral, and infrared sensors.
Types of Remote Sensing
Types of remote sensing can be classified according to various criteria reflecting the peculiarities of their application and technologies. One of the main methods of classification is based on the division by ranges of electromagnetic radiation, such as visible light, infrared and ultraviolet radiation, microwaves, and radio waves. Additionally, remote sensing types can be divided into active and passive, depending on whether the system uses its own source of radiation or detects reflected radiation. There is also a classification based on the type of platform on which sensing sensors are installed, such as satellites, UAVs, or aerostats. Each of these types has its own characteristics and provides valuable information for a wide range of applications, from monitoring climate change and the environment to urban development planning and agriculture.
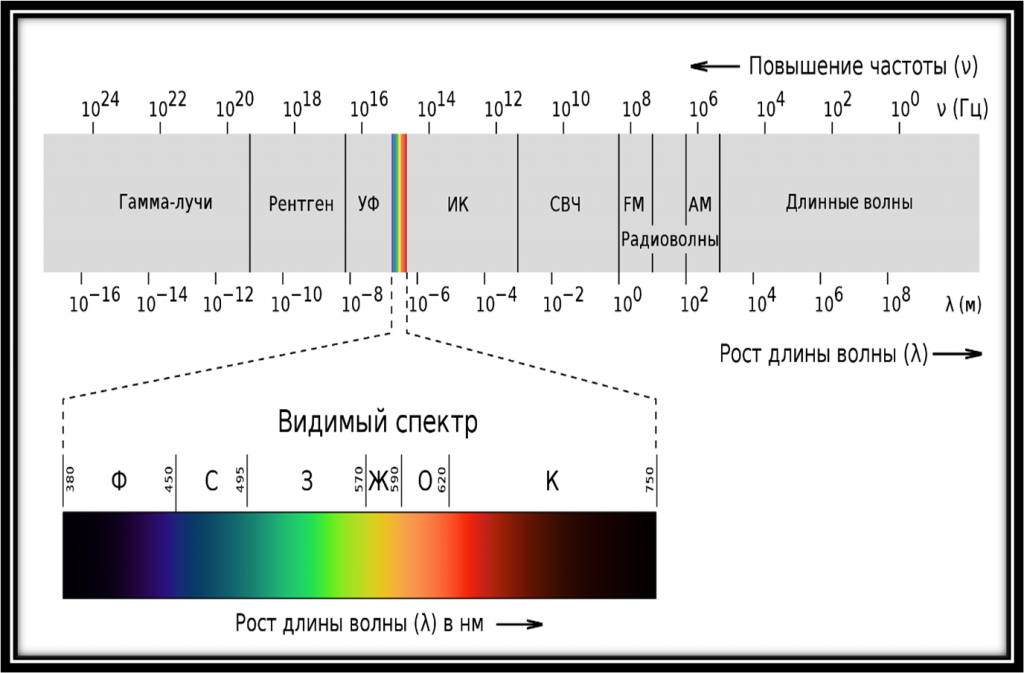
1. Optical Sensing:
- Features: Uses visible and infrared spectrum to obtain images of the Earth.
- Advantages: Provides high spatial resolution, allows for detailed surface imaging.
2. Thermal Sensing:
- Features: Measures thermal radiation from the surface to analyze temperature characteristics.
- Advantages: Allows for the detection of temperature changes, identification of hot spots (including oil leaks), useful for monitoring the thermal state of objects.
3. Radar Sensing:
- Features: Uses the radio frequency range of electromagnetic waves to image the surface, penetrates through clouds, and operates in various weather conditions.
- Advantages: Provides the ability to work in low-light conditions or adverse weather, detects changes in terrain and surface structure.
4. Hyperspectral Sensing:
- Features: Measures reflected or emitted electromagnetic radiation at discrete wavelengths across a wide spectrum with narrow bands.
- Advantages: Allows for obtaining spectral characteristics of objects across a broad spectrum, provides high ability to distinguish surface types and substances.
Classification by Spatial Resolution of Aerospace Imagery:
- Very Low (worse than 100 m).
- Low (15–100 m).
- Medium (5–15 m).
- High (1–2.5 m).
- Very High (0.3–0.5 m).
- Ultra High (0.02–0.5 m).
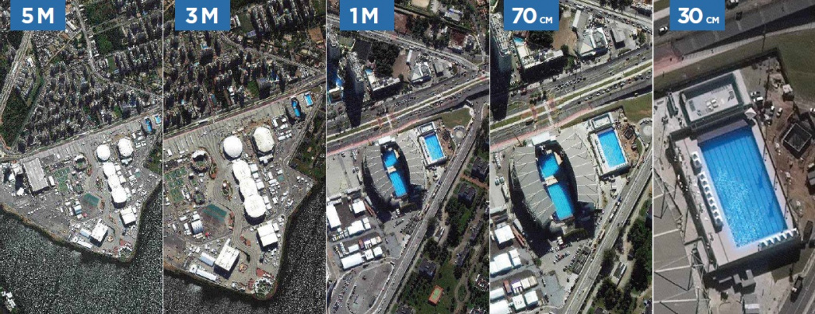
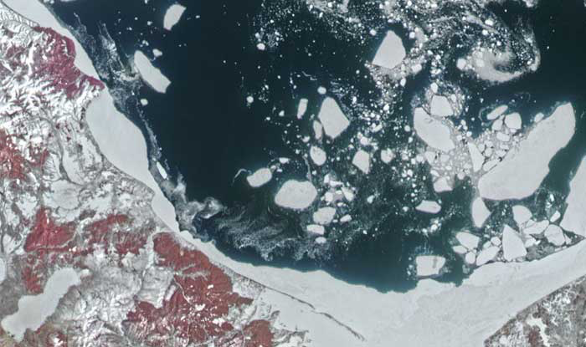
Average spatial resolution satellite imagery (10 m) is the most popular due to the availability of public portals and catalogs of images, such as US Geological Survey (USGS) and Sentinel Hub. Scientists and students from around the world use data from these portals for scientific research and practice in remote sensing.
However, engineering works require data with higher resolution (from 0.15 m to 1 m).
There are also other categories of remote sensing.
Passive Method
In passive sensing, measurements are made using radiation emitted from objects on the Earth's surface, thus not requiring the use of a radiation source in the required spectrum range.
Active Method
In active sensing, its own radiation source is used, which illuminates the Earth's surface, and then the signal returned from the objects is detected and analyzed.
For example, RADAR and LiDAR sensors are typical active remote sensing instruments that measure the time delay between emission and return to establish the location, direction, and speed of an object. The collected remote sensing data is then processed and analyzed using remote sensing equipment and computer software (the most complex solution being analytical products presented in near real-time), which are available in various applications, primarily in geographic information systems (GIS).
Types of Sensing:
1. Satellite Sensing:
- Includes the use of spacecraft to recognize Earth's surface features, weather conditions, and other parameters.
- Applications: used for a wide range of research, monitoring, mapping, and other applications.
2. Aerial Photography:
- Based on the use of a camera mounted on an aircraft, helicopter, or other manned vehicle to capture images of the Earth's surface from flight altitude.
- Application: used in geography, cartography, scientific conditions, and in searching for the human factor in wilderness areas.
3. UAV Imaging (Unmanned Aerial Vehicles):
- Specialized devices that allow for aerial photography without a person on board.
- Application: used in monitoring fields, forests, and industrial forest areas for safe and efficient problem recognition. It has the highest spatial resolution.
For example, generalized characteristics of the most demanded remote sensing data sources on the market today are shown in Table 1.
Parameters | Satellite | Aerial Photography | |
---|---|---|---|
Manned Aviation | Unmanned Aerial Vehicles | ||
Optimal Imaging Area | >100 km2 | 50-1000 | <100 km2 |
Sensors | Multispectral | Multispectral | Multispectral |
Hyperspectral | Hyperspectral | Hyperspectral | |
Thermal | Thermal | Thermal | |
Radar (Radiolocation) | Radar (Radiolocation) | Radar (Radiolocation) | |
LIDAR (Laser Scanning) | LIDAR (Laser Scanning) | LIDAR (Laser Scanning) | |
Spatial Resolution | 0.25 m to 10 m | 0.1 m to 1.5 m | 0.02 m to 1 m |
Cost of Image per Area (USD) | |||
1 km | - | - | 200 |
5 km | - | - | 500 |
25 km | 200 | - | 1,250 |
50 km | 500 | 6,250 | 1,750 |
100 km | 1,000 | 10,000 | 3,000 |
1000 km | 10,000 | 40,000 | - |
10000 km | 70,000 | 150,000 | - |
Problems to be solved
- Using remote sensing data in environmental research allows for a better understanding of various aspects including climate, atmospheric conditions, land surface, vegetation, hydrology, and others. These studies are important for monitoring changes in the environment and understanding its dynamics. Satellite data allows for tracking extensive ice caps and controlling the reduction of ice cover in the Arctic.
- Data obtained through remote sensing is used for weather analysis and forecasting, improving weather predictions, and enhancing people's safety. Accurate hurricane data allows for timely evacuation of populations from disaster-prone areas, iceberg tracking, and monitoring ice on the Northern Sea Route enables ships to transport cargo quickly and safely.
- Remote sensing enables the creation of high-quality maps and terrain models, which are necessary for urban development, agriculture, transportation infrastructure, and geodesy. For example, using satellite data in planning new road networks helps optimize traffic flow and reduce traffic jams.
- Monitoring and managing natural resources with remote sensing data efficiently optimize resource utilization, promptly prevent forest fires, control water pollution, and ensure sustainable environmental management. For example, monitoring forest areas allows for the prompt detection of fire outbreaks and firefighting before they spread.
- Remote sensing plays a crucial role in studying and monitoring climate change, allowing scientists to study changes in ice cover, sea level, surface temperature, and other factors related to climate change. For example, satellite observation data helps assess the rate of Antarctic ice melting and its impact on the global sea level.
- Monitoring and warning of natural disasters using remote sensing help to respond promptly to threats and organize rescue operations during earthquakes, volcanic eruptions, floods, and other disasters. For example, timely tsunami warnings save thousands of lives in coastal areas.
- Observing changes in ecosystems using remote sensing helps study forest degradation, loss of biodiversity, changes in species distribution, and various forms of ecological restoration. For example, monitoring the threat of extinction of certain animal species helps develop measures to conserve their populations.
- Monitoring anthropogenic impact using remote sensing allows tracking the influence of human activities on the environment, assessing air and water pollution, land use changes, and urban and infrastructure development. For example, analyzing greenhouse gas emissions data helps control atmospheric pollution levels and take measures for environmental protection.
Remote sensing applications and services are diverse and can be applied in various fields, including science, ecology, geography, agriculture, urban planning, transportation, and other industries.
Examples of Remote Sensing Data and Their Use
Panchromatic Data
Panchromatic data is obtained in the visible spectrum of electromagnetic radiation with wavelengths from 400 to 700 nm. These data are black and white images with high resolution, captured by one channel. Satellites such as WorldView-3, Pleiades, and QuickBird can provide panchromatic data with resolutions up to 30 cm on the ground. For UAVs, examples of devices include DJI Phantom 4 Pro, senseFly eBee X, WingtraOne with resolutions up to 2–5 cm on the ground. For aerial photography from helicopters or airplanes, Phase One iXU-RS1000 cameras with resolutions of 10–20 cm on the ground are used.
- Advantages:
-
- High spatial resolution for detailed terrain analysis.
- Wide range of applications in cartography, urban development planning, and environmental monitoring.
- Disadvantages:
- Limited information spectrum due to operation only in the panchromatic channel.
- Products:
- Orthophotos, digital elevation models, classified land use maps.
- Areas of Use:
-
- Geology: helps in detecting geological structures and mineral resources.
- Archeology: used to search for archaeological objects and monitor archaeological sites.
- Urban planning: allows monitoring changes in urban development and planning new infrastructure objects.
- Forestry: applied for monitoring forest areas, controlling logging, and timber harvesting.
- Landscape design: used for planning land improvement and creating unique landscapes.
Need for consultation?
Fill the form and we will contact you
Completed projects
Our clients
FAQ
Spatial data - any type of data that directly or indirectly relates to a specific geographic area or location. Often referred to as geospatial data or geospatial information, spatial data can also numerically represent a physical object in a geographic coordinate system.
However, the concept of spatial data is much more than just a spatial component, such as maps, for example.
It should be understood that there are raw data (which are 80% images) obtained from remote sensing sensors, processed data (remote sensing products, usually in GIS shells), and analytical data - information, clues for a particular industry, task, or military system.
There is a huge difference between data and information. Data are raw, voluminous, and often useless. On the other hand, information is power. Information is useful to the end user and helps make informed decisions that can be the difference between success and failure.
From the perspective of the geospatial industry, companies engaged in remote sensing analytics represent the difference between data and information. Using powerful algorithms, they can transform large volumes of data into information that is used by organizations of all sizes and shapes to make decisions.
Users can store spatial data in various formats, as they may contain not only location data. Analyzing this data helps better understand how each variable affects individuals, communities, population groups, territories, objects, etc.
There are several types of spatial data, but the two main types of spatial data are geometric data and geographic data.
Vector and raster (Fig. 1) are common data formats used for storing geospatial data.
Vectors are a graphical representation of the real world. There are three main types of vector data: points, lines, and polygons. Points help create lines, and connecting lines form closed areas or polygons. Vectors often represent the generalization of features or objects on the surface of the planet. For example, vector data (used by over 78% of users) is stored in shapefiles, sometimes called shp files (ArcGIS software).
Raster represents information presented in a pixel grid. Each pixel stored in the raster has a value. It can be anything from a unit of measurement, color, or information about a specific element. Typically, raster refers to images, but in spatial analysis, it often refers to orthoimagery or photographs taken and initially processed from aerial devices or satellites.
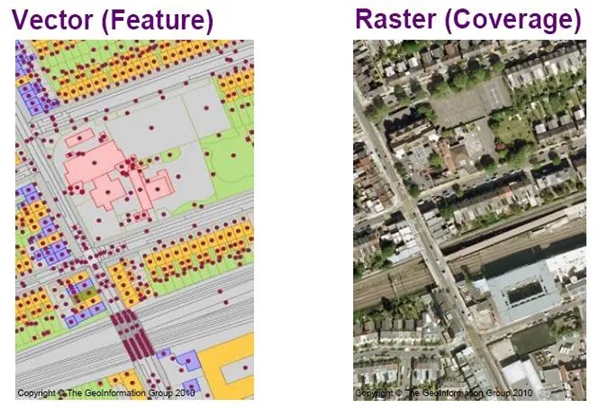
There is also something called an attribute. Whenever spatial data contains additional information or non-spatial data, they are referred to as attributes. Spatial data can have any number of location attributes. For example, this could be a map, photograph, historical reference, or anything else deemed necessary.
Spatial data technology focuses on extracting deeper insights from data using a full set of spatial algorithms and analytical methods. Modern methods include using machine learning and deep learning to uncover hidden patterns in data. This approach helps improve predictive models.
Spatial data can also include attributes that provide additional information about the object they represent. This helps users understand where things are happening and why. For example, Geographic Information Systems (GIS) and other specialized software applications help access, visualize, manipulate, and engage in spatial analysis.
Experts expect spatial data science to become more important as government agencies and businesses strive to make more informed decisions based on data.
Other aspects of spatial data science include spatial data intelligence analysis and data visualization.
Spatial data intelligence analysis describes the process of discovering hidden patterns in large spatial data sets. As a key factor in application development, GIS, spatial data intelligence analysis allows users and industry professionals to extract valuable data about neighboring regions and explore spatial patterns. This scenario considers spatial variables such as distance and direction.
Data visualization software allows connecting various spatial data files in geospatial technology, such as Esri File geodatabases in ArcGIS, GeoJSON files, Keyhole Markup Language (KML) files, MapInfo tables, shapefiles, and TopoJSON files, among others.
After connecting, GIS specialists or users can create point, line, and polygon maps using information from spatial data files, lidar data files, and geospatial data files.
Over the past 20 years, spatial data has been used not only for mapping (the share of map creation from remote sensing data in 2021 is 60%, compared to 95% in 2000) but also for new directions such as BIM, SMART, IoT, Outdoor/Indoor.
Navigation technologies, mobile technologies form the fundamental basis for spatial data. For example, popular mobile applications allow data developers to create complex combined applications using sets of geospatial and temporal data from IoT data, maps, weather, UAVs, satellites, etc.
Today, spatial data occupies a leading niche in technology for managing and selecting the necessary information component in the BIG DATA environment, machine reading (artificial intelligence), neural network analysis, and other promising areas of science and IT technologies.
Practically, the ecosystem of human habitat and development is related to spatial data - land, water, ocean, atmosphere, nature, resources, cities, roads, any infrastructure, social, political, and military interactions.
Quality and technical characteristics of Earth remote sensing sensors, as well as the conditions of Earth remote sensing acquisition, are determined based on their spatial, spectral, radiometric, and temporal resolutions (acquisition frequencies), which are determined by the task for data processing and obtaining the final product or service of Earth remote sensing.
Customer tasks determine the selection of sensor data characteristics.
Spatial resolution
The size of a pixel recorded in a raster image. Usually, pixels can correspond to square areas with sides ranging from 0.15 to 1000 meters.
The ability to capture the Earth's surface in one pixel, for example, of satellite sensors, is called spatial resolution (commonly referred to as ground resolution). In some cases, spatial resolution depends on the orbit on which the satellite flies.
Currently, great attention is paid to increasing the spatial resolution of satellite sensors. It reaches 15–25 cm. Increasing spatial resolution improves image quality.
Aerial and UAV imagery has resolutions of up to 3–5 cm, and ground lidar imagery has resolutions of up to 3–5 mm.
Visual comparative images for understanding spatial resolution are shown in Fig. 2, 3, 4, 5.
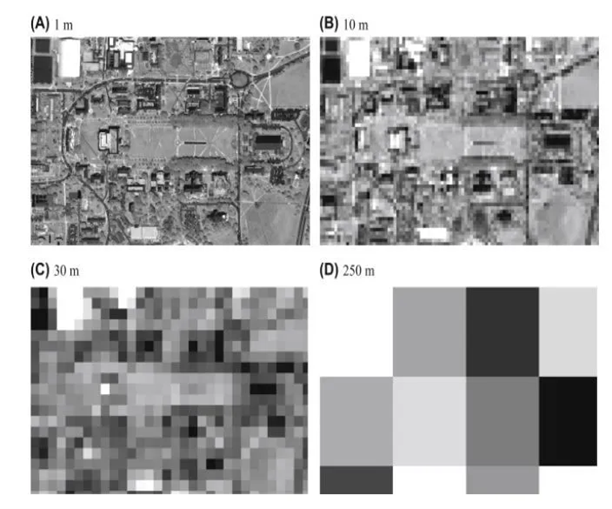
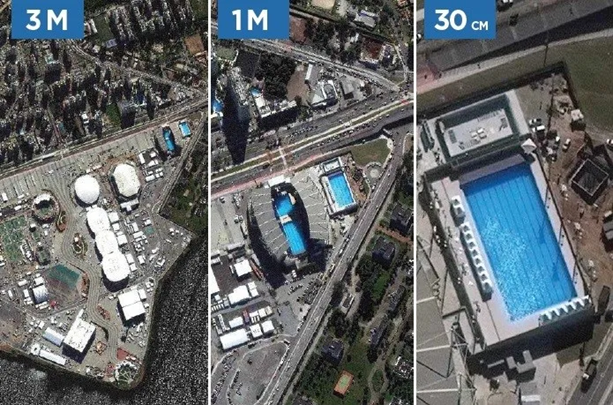
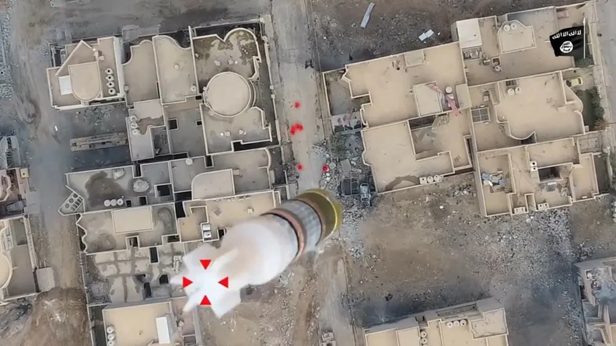
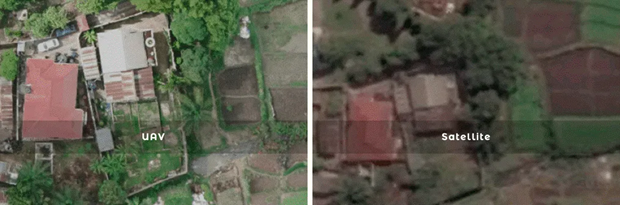
Spectral resolution
The wavelength of different recorded frequency ranges—usually related to the number of frequency ranges of the electromagnetic spectrum recorded by the sensor platform (Fig. 6).
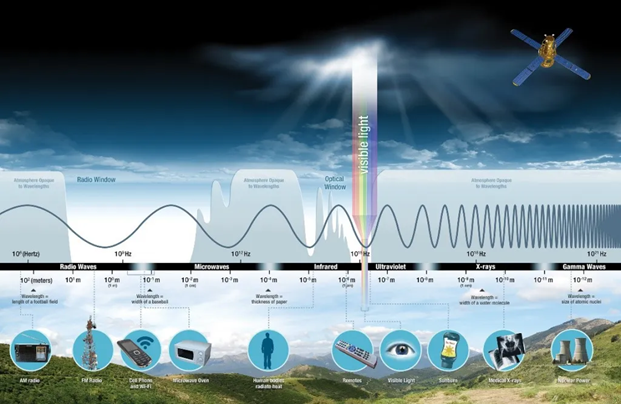
Spectral resolution is a sensor characteristic that captures images in different wavelengths of the spectrum. Modern remote sensing utilizes panchromatic, multispectral (Fig. 7), hyperspectral, and super spectral images based on this characteristic. Different objects reflect rays differently in different wavelengths. Therefore, to determine any object characteristic, it is necessary to study its reflective properties in different spectrum wavelengths. For example, to use an image as a background, it is sufficient to have its image taken in three spectrum ranges: green, blue, and red (RGB).
However, if we want to obtain more data from it, for example, to express impermeable surfaces, vegetation classification, we should use the near-infrared (NIR) or thermal IR. In this case, the capabilities of the MODIS scanner are not limited. It captures images in 36 spectra ranging from 0.4 μm to 14.4 μm and transmits them to Earth. The more ranges, the easier it is to identify an object. In this regard, the Landsat 8, 9 satellite scanner ranks second. Its spectral image is taken in 10 ranges (coastal, blue, green, red, NIR, SWIR1, SWIR2, Pan, TIR1, TIR2). Taking into account the identification of water atoms in the Cirus band as one band, the number of Landsat 8 OLI TIRS bands reaches 11 bands. The point is that the Cirus band does not capture images in the light spectrum of wavelengths but operates according to the function of light return of oncoming aerosols. The last place is occupied by the WorldView2 and WorldView3 satellites. The sensors of these satellites transmit images to us in 9 spectral wavelength ranges. These ranges are Coastal, Blue, Green, Yellow, Red, RedEdge, NIR1, NIR2, and Pan. For this reason, MODIS satellite scanners received a high rating. But due to poor spatial resolution, it is not widely used.
Therefore, currently, the highest demand is for data from the WV3 satellite. Especially its extended red range.
Thematic solutions for space data create most of the Earth remote sensing products based on WV3 images.
The conditions for the normal operation of sensors, i.e., obtaining quality images, are determined by the atmospheric transparency windows, allowing the passage of a specified section of the sensor's electromagnetic spectrum.
The spectral characteristics and atmospheric transparency windows for Earth remote sensing sensors are presented in Fig.7.
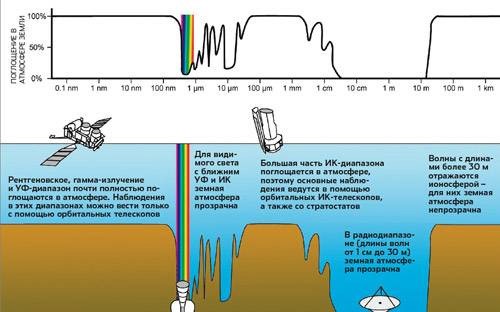
Radiometric resolution
The number of different radiation intensities that the sensor can distinguish. Usually, this ranges from 8 to 14 bits, corresponding to 256 levels of the grayscale and up to 16,384 intensities or "shades" of color in each band. This also depends on the sensor's "noise."
The difference in radiometric resolution is easy to visualize in Fig. 8
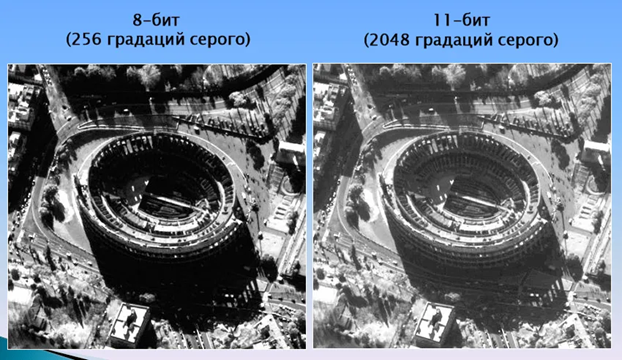
Temporal resolution
The frequency of satellite or aircraft (UAV) passes and image acquisition over the same territory or observation object. It is of great importance for studies of time series of Earth remote sensing data when monitoring processes (agriculture, construction, military affairs, water resources, emergencies, etc.). For example, satellite temporal resolution of Earth remote sensing today in military affairs reaches 15 minutes, and in civil affairs, 1–2 hours, and seasonal resolution can be annual (archive data). Archive data for in-depth study of territory changes can be ten years (Fig. 9, 10).
For most systems, such as space remote sensing, it is assumed to extrapolate sensor data relative to a ground control point, including distances between known ground points. This is called a reference point, which is used to then correct the image, map, or diagram.
Therefore, image processing technology, photogrammetry, is of great importance.
Additionally, radiometric and atmospheric correction of images may be required.
Radiometric Correction
Allows avoiding radiometric errors and distortions. The illumination of objects on the Earth's surface is uneven due to different terrain properties. This factor is taken into account in the radiometric distortion correction method. Radiometric correction sets the scale of pixel values; for example, a monochromatic scale from 0 to 255 will be transformed into actual brightness values.
Topographic Correction (also called terrain correction)
In rugged mountains, effective pixel illumination varies significantly due to terrain relief. In remote sensing imagery, a pixel on a shaded slope receives weak illumination and has a low brightness value, unlike a pixel on a sunny slope receiving strong illumination and having a high brightness value. For the same object, the brightness value of a pixel on a shaded slope will differ from that on a sunny slope. Moreover, different objects may have the same brightness values. These ambiguities seriously affected the accuracy of information extraction from remote sensing images in mountainous areas. This became a major obstacle to further application of remote images. The goal of topographic correction is to eliminate this effect, restoring the true reflectance or brightness of objects under horizontal conditions.
Atmospheric Correction
Elimination of atmospheric haze by rescaling each frequency band so that its minimum value corresponds to the pixel value of 0. Digitizing data also allows manipulating data by changing gray scale values (Fig. 12).
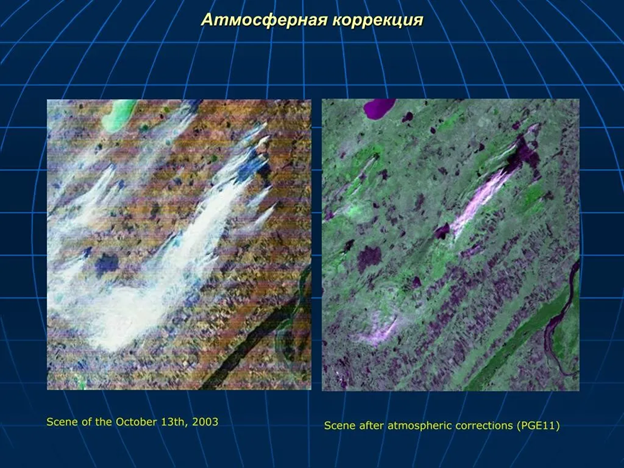
There is a rule to provide meteorological conditions for the date and time of Earth remote sensing acquisition.
Levels of Earth remote sensing data processing were first defined by NASA in 1986. Their definition and image processing technology are used in global Earth remote sensing standardization, with changes and approvals made in the United Nations.
Primary data processing (from raw images to their georeferencing) of Earth remote sensing data is at levels 0 to 3. This is followed by the so-called thematic processing. There is a classification of thematic processing that exceeds more than 250 directions. The thematic scope is supplemented every year. Thematic technology is tailored to the Customer's task. Currently, the main product of Earth remote sensing is analytics.
The most requested product of Earth remote sensing is military affairs, agriculture, and construction.
When planning and implementing Earth remote sensing projects, it is important to consider several key parameters:
- Size of the area of interest
- Accessibility of the area for imaging
- Timeframe for imaging
- Whether the imagery is archival or new
- Spectral channels
- Project budget
1) Speed and simplicity of data collection: Satellites are capable of covering thousands of square kilometers in a matter of minutes. This allows for collecting a large volume of information in a short period of time without the need for complex flight planning, obtaining permissions, and selecting take-off and landing points for aircraft. Thus, satellites have high speed and simplicity of data collection.
2) Absence of logistical problems: Satellites do not encounter problems associated with airspace restrictions, complex route planning, and constantly changing restrictions on the survey area. They can collect data from isolated, conflicting, or transboundary locations without limitations, which is especially valuable for large-scale cartographic projects.
3) Weather independence: Unlike aviation methods, which are limited by weather conditions, satellites can operate independently of weather, except for cloud cover. This ensures the continuity of data collection and reduces the risks of delays or cancellations of surveys due to weather conditions.
1) Resolution and spectral characteristics of sensors: Customers set requirements for spatial resolution and spectral ranges that correspond to their specific needs. This allows obtaining data with the required level of detail and spectral information for the phenomena or objects under study. As of May 2023, the choice of Earth observation satellites is vast.
2) Viewing angles: Customers specify viewing angles to capture images from optimal perspectives. This is particularly useful for analyzing three-dimensional objects or terrain, where specific angles can provide a more comprehensive understanding and analysis.
3) Real-time weather updates: Regular real-time weather updates help avoid capturing images under unfavorable weather conditions and clouds. This allows maximizing the use of available windows for imaging.
4) Fast data delivery: After capture, satellite images are uploaded via ground stations and can be delivered to the user within 4 hours. This ensures prompt access to fresh data and enables quick analysis and processing.
5) Data processing and delivery methods: Users can choose from various data processing options according to their requirements.
Operators of Earth observation satellites offer a wide range of imaging capabilities, providing various spectral ranges and ultra-high resolution.
1) Spectral ranges: Satellite operators offer images in different spectral ranges. This allows analyzing objects and phenomena at different wavelengths and obtaining specific spectral information. Hyperspectral imaging can cover hundreds of spectral ranges, expanding research possibilities.
2) Stereo imaging: Satellites can also perform stereo imaging, allowing for stereo images from different angles. Stereo images provide reliable data for creating Digital Elevation Models (DEM) and virtual 3D models, which is useful for terrain analysis and three-dimensional objects.
3) Ultra-high resolution: Satellites provide high-resolution images. For example, Spacewill's Superview NEO satellite can offer satellite images with resolutions up to 30 centimeters. This allows identifying various objects and features on the ground with high accuracy and detail.
1) Accessibility and predictability: Satellites can reach areas that may be difficult or inaccessible to other imaging methods, such as remote or geographically isolated locations. Thanks to Earth observation satellite constellations, terrain imaging plans become more accessible and predictable for clients.
2) Refresh rate: High refresh rates of satellite imagery allow for obtaining fresh data at regular intervals. This is particularly important for automated analysis, where constant data updates are required. Users can confidently rely on the continuous availability of data for their workflow processes. As of May 2023, GEO Innovator LLC offers its clients satellite imaging from 100+ Earth observation satellites with resolutions better than 1 meter.
3) Integration with artificial intelligence programs: Satellite images can be integrated into programs utilizing artificial intelligence (AI) for automatic object extraction and classification on the imagery. This helps optimize workflow processes and automate data analysis.
4) Expanded training data: With a large amount of imagery collected by satellites over time, users have access to expanded training data for machine learning programs. This enables improving the quality and accuracy of machine learning models used for satellite data analysis.
5) Historical data and modeling: Satellite data provides access to historical data that can be used for modeling and forecasting. This is particularly important for trend analysis, anomaly detection, and profitability assessment. Utilizing historical data helps understand long-term changes.
These satellite imaging capabilities ensure high precision and data quality, making them an effective alternative to aerial imaging. As of May 2023, there is a global trend towards the displacement of manned aviation by unmanned aerial vehicles at a rapid pace.
GEO Innoter LTD offers its clients integrated solutions combining satellite and UAV technologies.
Distant sensing of the Earth (DSE) is a method of studying the Earth and its environment, in which information is gathered using satellites or other remote devices located at altitudes above the Earth's surface.
DSE allows for the collection of a wealth of data about objects on the Earth's surface, including geological structures, landscapes, climatic conditions, and other parameters. This method is used in various industries, including geology, geography, agronomy, ecology, meteorology, hydrology, and so on.
In geology, distant sensing of the Earth can be used to search for deposits of valuable minerals, including oil and gas, as well as to study geological structures, such as folds, fractures, etc.
With the help of distant sensing of the Earth, changes on the Earth's surface caused by various factors, such as climate change, natural disasters, or anthropogenic impacts, can also be studied.
In general, distant sensing of the Earth is a powerful tool for studying the Earth's surface and its environment, and it finds wide application in various scientific and practical fields.
There are numerous satellites in space that are used for Earth remote sensing data acquisition. They can vary in purpose, orbit characteristics, resolution, spectral characteristics, and other parameters. Let's consider the most common types of satellites for remote sensing:
- Optical satellites - used for obtaining information in the visible and infrared ranges. Examples of such satellites include Landsat, Sentinel-2, SPOT, MODIS.
- Radar satellites - utilize radio wave radiation to obtain data. They can operate in any weather conditions and during daytime. Examples of such satellites include RADARSAT, Sentinel-1, TerraSAR-X.
- Gravitational satellites - used for measuring the Earth's gravitational field and the mass of objects on its surface. Examples of such satellites include GRACE, GRACE-FO.
- Geodetic satellites - used for precise determination of coordinates and heights of points on the Earth's surface. Examples of such satellites include GPS, GLONASS, Galileo.
- Atmospheric satellites - used for studying atmospheric phenomena such as clouds, atmospheric gases, meteorological conditions. Examples of such satellites include Aqua, Terra, MetOp.
- Space telescopes - used for studying celestial objects and phenomena such as stars, galaxies, cosmic dust. Examples of such satellites include Hubble Space Telescope, Chandra X-Ray Observatory, Spitzer Space Telescope.
Each of these types of satellites has its own characteristics and applications, and the choice of a specific satellite depends on the task that needs to be addressed.
Remote sensing of the Earth provides a wide range of capabilities for obtaining information about the Earth and its surface from space. Some of the key capabilities of remote sensing include:
- Obtaining information about the geographical location of objects on Earth. With satellite remote sensing, precise coordinates and elevations of geographical objects such as mountains, rivers, lakes, populated areas, etc., can be obtained.
- Studying changes in the natural environment. Remote sensing enables monitoring changes in forested areas, pastures, as well as other natural environments such as deserts, tundras, etc. This allows tracking processes like erosion, deforestation, droughts, and other natural phenomena.
- Researching climatic processes. Remote sensing data is used to study climate changes on the planet, such as global warming, glacier retreat, etc.
- Monitoring and forecasting natural disasters. Remote sensing allows monitoring processes related to earthquakes, volcanic eruptions, floods, and other natural disasters. This enables forecasting hazards to the population and taking measures to prevent natural disasters.
- Exploration and extraction of natural resources. Remote sensing enables the discovery of deposits of natural resources such as oil, gas, gold, silver, etc. This is especially useful in remote and underexplored areas.
- Monitoring environmental pollution. Remote sensing data is used to monitor pollution of water and air resources, as well as to monitor soil quality, etc.
- Investigating oceans and seas. Remote sensing provides information on temperature, salinity, currents, waves, and other parameters of oceans and seas. This allows forecasting conditions for fishing, tracking iceberg movement, predicting sea level changes, and other parameters important for studying marine ecosystems.
- Observing transportation and communications. Remote sensing enables tracking the movement of vehicles such as cars, trains, ships, and aircraft. This can be useful for controlling traffic flows and optimizing routes. Remote sensing data can also be used to detect oil or gas leaks in pipelines and other communications.
- Supporting defense and security. Remote sensing is used to ensure state security by monitoring borders, controlling land and air surfaces. Additionally, remote sensing can assist in detecting sources of terrorist threats and preventing possible attacks.
- Observing celestial objects. Remote sensing data is used to study celestial objects such as planets, galaxies, stars, etc. With remote sensing, their properties, physical characteristics, and movements can be studied.
In general, remote sensing allows obtaining information about the Earth and its environment, which can be used for decision-making in various fields of activity, from economics to ecology and science.