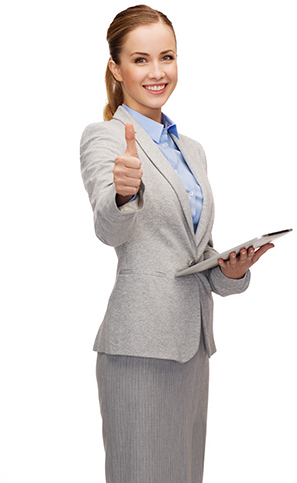
You can order from us
Prices for services
Consultation | Free |
---|---|
Preliminary analysis | Free |
Aerospace imaging | The cost of remote sensing materials is calculated individually for each order and may vary. Minimum cost starts from $0.5 per 1 km2. |
Execution time | Execution of a new imaging task takes at least 5 working days from the moment of prepayment. Execution time may be extended for significant areas and challenging climatic conditions in the area of interest. Delivery of archival data takes approximately 3 days. |
The cost of the aerospace imaging depends on the area size, quality requirements, and the type of the final product, such as orthophotoplan, CMM, CMR, 3D model, the need for thematic processing, etc. The cost is calculated individually for each customer.
The cost of execution is calculated on an individual basis, taking into account a specific of task.
After receiving the task description, we calculate the cost and send you a commercial offer.
Period of execution
Agreement on requirements for remote sensing materials: 1 to 5 days*
Contract signing: 1 to 5 days*
Ordering the imaging task (task assignment to the satellite operator): 5 days**
Receiving archival remote sensing materials: 3 days**
Thematic processing of remote sensing materials (if necessary): 15 days*
TOTAL TIME: 15 days
* working days
** from the date of receiving 100% advance payment
The timeline for aerospace imaging depends on the total area of the territory, imaging requirements, and the final product. It is calculated individually for each customer.
How to place an order:
Need for consultation?
Fill the form and we will contact you
Stages of service provision
The result of the provision of services
Creation of the final product based on the imaging materials:
- Archival images of various types: black and white, color, multispectral, synthesized over the monitoring period, according to the Client's requirements.
- Materials from new imaging of various types.
- Thematic LULC maps.
- Results of LULC analysis in agreed-upon indices and formats.
GEO INNOTER provides the Client who requested the imaging materials with the final product according to the Technical Task on electronic media or via the Internet through FTP servers.
Requirements for Source Data
Accurate coordinates of the area of interest, precise requirements for remote sensing materials (spatial resolution, imaging type, maximum image tilt angle, minimum solar angle, imaging period), additional requirements for the final product (if necessary), output data formats.
If it is not possible to provide the specified information, provide information about the intended use of the remote sensing materials, and the specialists of GEO INNOTER will analyze the requirements and propose the optimal solution.
Related services
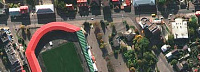
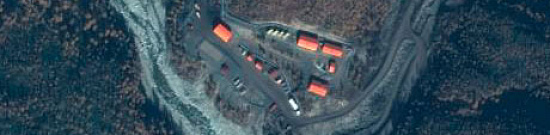
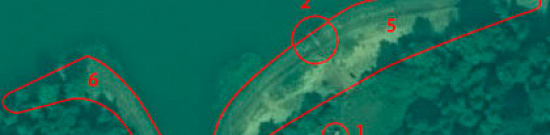
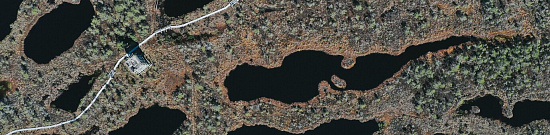
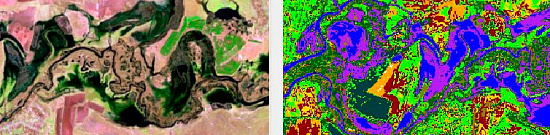
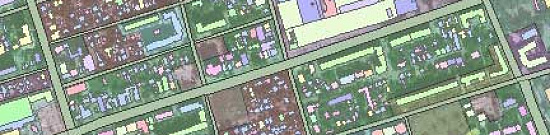
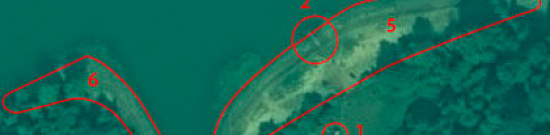
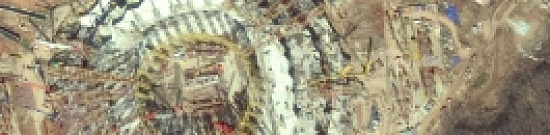
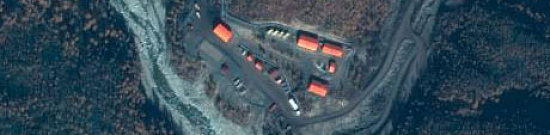
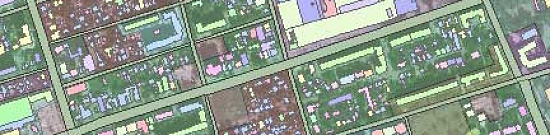
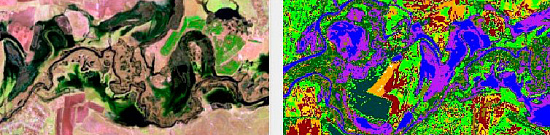
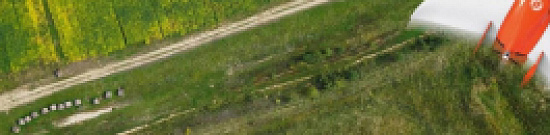
Completed projects
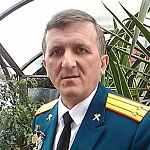
Customers
FAQ
- The task that needs to be addressed using the images;
- Area of interest (location / coordinates of the object in any convenient format, and the area of the object);
- Date or time interval for which archival imagery can be considered or new imagery can be acquired;
- Requirements for the imaging (image tilt angle, solar angle, spatial resolution, imaging type, cloud cover, tolerance for snow cover).
- As a rule, the minimum area for ordering archival satellite images is 25 km2, and for a new satellite survey, it is 100 km2. The minimum strip width (distance between two closest points) depends on the satellite operator, survey mode, and product, and can range from 2 to 5 km.
Selected examples:
- Urban or developed land (Residential / Commercial and other services / Industrial areas / Communications and utilities / Mixed urban or developed land)
- Agricultural land (Arable land and pastures / Gardens, groves, vineyards, nurseries and ornamental garden plots)
- Grassland (Grassland / Shrub and bush pasture / Mixed pasture)
- Forest fund lands (Deciduous forests / Evergreen forest land / Mixed forests)
- Water Resources (Rivers / Streams and Canals / Lakes / Reservoirs / Bays)
- etc.
Licenses
Warranty
We guarantee 100% quality of services. Cooperating with GEO Innoter specialists, you exclude risks and losses!The availability of qualified personnel able to work with specialized equipment, software and experience allows us to provide these guarantees!